Project Aims to Revolutionize Design in Wind Engineering
Combined Cyber-Physical Optimization Approach Will Speed Up the Design Process
Published on July 31, 2017
University of Florida wind engineering class visits with researchers in the boundary-layer wind tunnel (BLWT).
Researchers at the University of Maryland and University of Florida are collaborating on a project to develop a cyberphysical systems approach to optimal design in wind engineering. The principal investigator on the project is Brian Phillips, assistant professor of Civil and Environmental Engineering at the University of Maryland.
Cyber-physical systems link the real world to the cyber world, using digital computers to monitor and control physical attributes in real time, Phillips explains. This application of cyber-physical systems combines the accuracy of physical wind-tunnel testing with the ability to efficiently explore design alternatives using numerical optimization algorithms. Through actuation, he says, the specimen in the wind tunnel morphs to create candidate designs. Because the specimen (model) is undergoing physical changes as it approaches the optimal solution, this approach is referred to as loop-in-the-model testing.
Pioneering Work
Traditional trial-and-error design approaches are inefficient. They require extensive iterations between architects and structural engineers, Phillips says. Our pioneering work will combine wind tunnel testing with computer-augmented design to produce optimal structural designs faster and with greater confidence than purely experimental or purely computational methods.
The research team has designed an objective and fully automated approach for the boundary-layer wind tunnel (BLWT) at the University of Floridas NSF-sponsored Natural Hazard Engineering Research Infrastructure (NHERI) Experimental Facility. Cyber-infrastructure, including a highperformance computer (HPC), was added to a traditional wind tunnel to coordinate turntable control, specimen actuation, data acquisition, data analysis, and the execution of scripted optimization algorithms.
Anticipated outcomes include:
- The combination of accurate experimental testing and numerically driven optimization for wind engineering.
- The advancement of optimization in a practical engineering setting.
- The discovery of new design and detailing features to achieve costeffective structures under wind loads.
A low-rise building model with a parapet wall of variable height was created as a proof-of-concept for the optimization approach. The sharp edges on bluff bodies, in particular the windward roof edges on low-rise structures, cause a separation of the boundary layer and generate vortex flow with large suction loads, Phillips explains.
Parapet walls reduce these suction loads, preventing roof gravel and other loose material from becoming wind-borne debris that can damage a buildings envelope and lead to wind and rain intrusion. In the wind tunnel, the parapet height of a building model is adjusted using servomotors to create and evaluate candidate designs guided by heuristic search algorithms. The objective is to mitigate extreme roof pressures.
This proof-of-concept, completed in February 2017, was the first cyberphysical optimal design in wind engineering.
![]() |
![]() Roof suction
|
BLWT model with no parapet wall, 45° approach wind angle, and a qualitative distribution of extreme roof suction.
![]() |
![]() Roof suction
|
BLWT model with a 1 inch parapet wall, 45° approach wind angle, and a qualitative distribution of extreme roof suction.
Ongoing Experiments
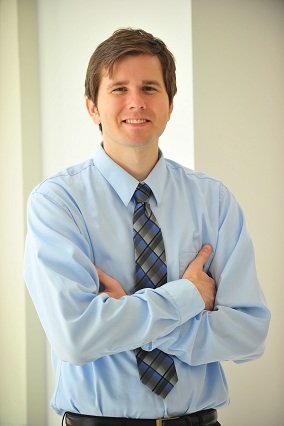
Brian Phillips is assistant professor of Civil and Environmental Engineering at the University of Maryland.
Currently, the team is preparing a series of tests to optimize the structural members carrying the wind load on the low-rise building model with adjustable parapet. Envelope wind pressures measured in the wind tunnel will be used as input to a finite element model of the structure.
The goal is to minimize user-specified objectives (e.g., material use) while ensuring strength and serviceability requirements constraints are met (e.g., code requirements for drift and acceleration).
Next Steps
The next phase of the project involves the consideration of aeroelastic models with multiple design variables. Aeroelastic models enable accurate physical modeling of fluid-structure interaction, useful when buildings dynamically respond to wind action (e.g., slender high-rise buildings, longspan bridges). The benefits of a cyberphysical approach to design will be clearly shown for a structure where the optimal solution may not be obvious and cannot be determined with traditional experimental or computational methods
This project is funded by NSF award #1636039, and it leverages the BLWT and HPC resources of the University of Florida NHERI site, supported by NSF award #1520843. Co-PI is Forrest Masters, professor and associate dean for Research and Facilities at the Herbert Wertheim College of Engineering, University of Florida.
NHERI Quarterly
Summer 2017
NHERI Five-Year Science Plan Published
Summer Updates from the NHERI NCO
The Importance of Data Publishing with Ellen Rathje
Project Aims to Revolutionize Design in Wind Engineering
Design Guidelines for Roof Pavers Against Wind Uplift
Hazard Researchers Embracing Biogeotechnics